
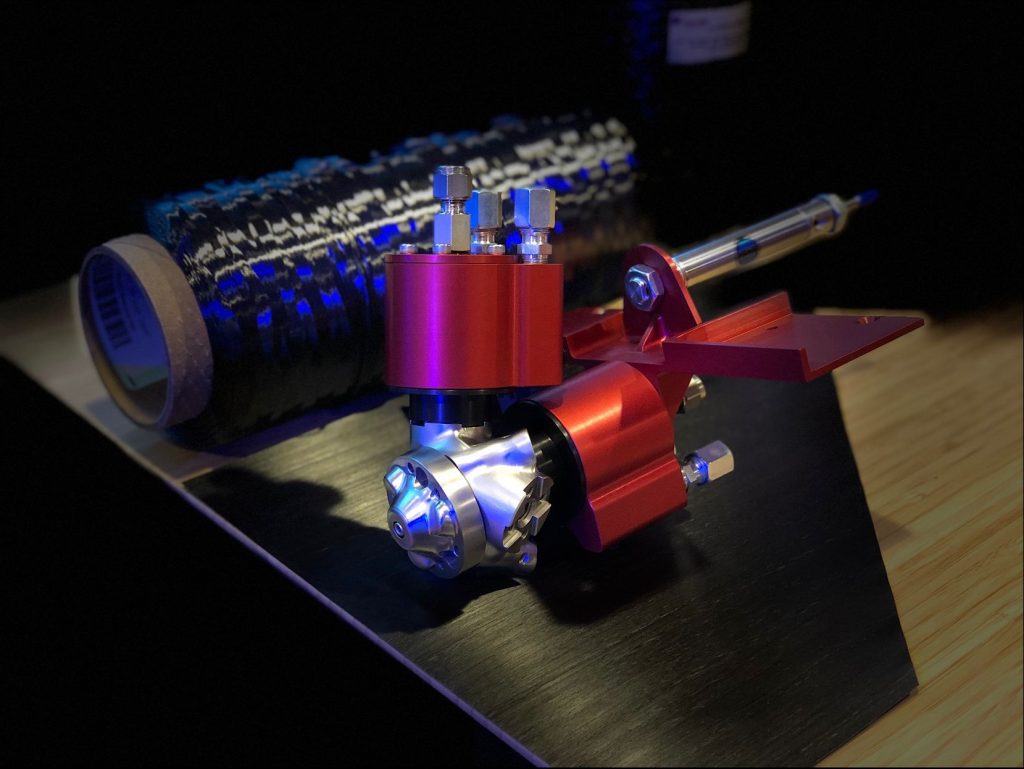
Faster Prints, Lower Costs
Additive manufacturing (AM) today is viewed by the composites industry primarily as a faster and less-costly method of producing prototype and low-volume composite parts. That perception is changing, however, as companies explore new techniques that will enable competitively priced 3D parts production on a much larger scale.
The industry’s growing interest in AM stems in part from the increased availability of desktop and medium-sized industrial 3D printers, according to Rick Neff, an AM technical and marketing consultant. Companies are experimenting with these printers, which typically combine carbon, glass or even aramid fibers (and sometimes tapes) with thermoplastic or photo-cured polymers.
At the same time, the technology for large-scale additive manufacturing (LSAM) has advanced. Thermwood Corporation, for example, has built upon the basic concepts of the Big Area Additive Manufacturing (BAAM) machine developed by Oak Ridge National Laboratory (ORNL) and private industry partners. Thermwood’s LSAM machines use a continuous cooling process to ensure each printed layer is at the optimal temperature to accept the next layer. This produces composite tools with vacuum integrity. “Other processes haven’t been good enough to create a tool without some sort of additional step to try to seal the tool or put a different surface on it that can hold a vacuum,” Neff explains.
In 2014, BAAM’s largest printed part was six feet long, 20 feet wide and eight feet high; Thermwood’s 1540 LSAM machine can produce parts 40 feet long, 15 feet wide and five feet tall and at a much faster speed. That’s led to new opportunities in the fast production of larger tools for aerospace and marine manufacturers.
“When we look at the cost of developing a prototype airplane, a large part of both the cost and the lead time can be attributed to tooling,” says Neff. “If it takes you months to get tools, it takes you months to build a plane. But if you can get tools in a week or two that revolutionizes the whole process of prototyping an airplane.”
Neff also notes that materials suppliers have developed a wider range of products specifically designed for AM. A manufacturer using an injection molding process can tolerate material shrinkage, which makes it easier to remove a part from a mold. But manufacturers don’t want a printed part to shrink, so they add carbon fiber or glass fiber to lower the coefficient of thermal expansion by a factor of 10, or an order of magnitude. “When you print parts, the hot layer on top doesn't shrink a lot and cause the part to warp and change its shape,” he says.
Construction Applications
Neff believes that advances in additive manufacturing, especially LSAM, will provide new opportunities in construction markets. While there’s a lot of hype around 3D printing concrete for buildings, he believes the real innovations – and the better economic proposition – is in printing composite forms for casting concrete.
Architects have discovered the potential of employing 3D-printed composites to create unique shapes for artwork or for retail store displays and museum exhibits. One example is the Al Davis Memorial Torch at the Las Vegas Raiders’ stadium, created from 225 composite material blocks that were 3D-printed with carbon fiber.
Architect Platt Boyd founded Branch Technology because he was frustrated with the constraints of traditional construction methods and materials. Inspired by natural forms and structures, Boyd found a way to incorporate those elements into buildings using the design freedom made possible by robotic, free-form 3D printing.
“We have a proprietary extrusion mechanism and proprietary algorithms that guide the robot through the path,” says David Goodloe, Branch Technology’s program development manager. “The material actually solidifies in free space as extruded, allowing us to print as if the robot was a giant pen tracing a three-dimensional path through the air and leaving behind a reinforced polymer in its wake.”
The result is volumetric lattice structures that have strength similar to comparable solid forms but use 20 times less material to build. If additional strength is needed in one area of the structure, the size of the honeycomb cells can be varied to increase the structure density in that location. Branch Technology works with a palette of materials that includes pelletized thermoplastic polymer resins with chopped carbon fiber and various additives.
The company currently has 14 robots, 12 for printing and two for milling. “Each one has its own build envelope, so we upload a specific part to a specific robot, and it prints that part within its own designated work cell,” explains Goodloe. Although the robot can print parts 30 feet long, 10 feet wide and 12 feet high, most parts range around 15 x 8 x 8 feet. Goodloe notes the size of the composite parts is usually constrained by logistical choke points such as a doorway or a truck, not by the printing technology.
Branch has three products. Branch Matrix™ is the exposed lattice structure of polymer resin and fiber additives. “It’s for architectural and sculptural applications – big, sweeping geometries,” says Goodloe. Nature Clouds, four giant hanging gardens in the center hall of Chicago’s Field Museum of Natural History, were printed by the company.
BranchClad™ is a mass-customized, ventilated rainscreen system and building skin that attaches to the structure of a building. Made with a lattice structure and a fire-rated, energy-efficient infilling foam, it enables the construction of unique exteriors. For a bank in Chattanooga, Tenn., Branch manufactured BranchClad panels with a wave pattern reminiscent of the waves on the bank’s logo. The company is currently working on cladding for the U.S. Space and Rocket Center in Huntsville, Ala., which will replicate the topology of the moon.
In partnership with Sto Corp., a prefabricator of construction components, Branch recently introduced StoPanel® 3DP, which provides builders with a wall assembly fully finished on both the exterior and interior sides.
Branch’s technology may be headed for space. The company is working with NASA to develop automated construction technologies for the moon and Mars. “The challenge is to print with in-situ materials to create habitats on these new worlds,” says Goodloe.
A Lego® Model for AM
The explosion of 3D-printed motor mounts for a drone launched Cole Nielsen, founder and CTO of Orbital Composites, on a quest to rethink additive manufacturing. To start, he spent 18 months studying every manufacturing method used today, with a special emphasis on advanced composites and sequential process compatibilities. Fundamentally, he found that carbon fiber, copper wires and electrically insulating polymers could monolithically create most of a vehicle system for any environment, but only if fibers were placed independently and arbitrarily. The matrix-to-reinforcement ratio would also need to be non-constant.
Nielsen developed a new type of printer head, the coaxial extruder. A nozzle within a nozzle, it encases a filament, such as continuous carbon or glass fiber or copper wire, inside a tube of thermoplastic or thermoset resin. This technology permits the inclusion of a variety of materials in the printing process. For example, a fiber optic cable introduced in the inner nozzle can be placed in the desired location on the part while simultaneously being encased with glue to hold it in place.
Nielsen also invented a high-force, high-pressure filament driver that enables faster printing and reduces filament failures. He notes that most failures in polymer extrusion printers are due to “snakebites” or filament drive failures.
Orbital Composites doesn’t use a conventional 3D printer with a gantry. Instead, the system employs single and multi-robot setups, with smaller robots stacked on top of larger robots. Their different end effectors perform specific tasks, such as printing and pre- or post-processing. The robots can work together in overlapping motion spheres, speeding production. They can easily print onto curved shapes and, unlike 3D printing systems that use gantries, the robots can manufacture products nine times their size. If a robot breaks down during the manufacturing process, another can take its place. “The robots can fail, or they come and go, but the object remains in the manufacturing process,” Nielsen says.
The company designs each printer setup around the product it’s producing. Nielsen likens it to putting together different Lego blocks to achieve a desired shape.
While this hardware is important, it’s only part of the story. “Fifty percent and maybe even more of our engineering effort is software. One of the ways that we use brute-force machine learning is to try to figure out how the robot needs to move to finish the print – literally how many different ways you can try to get through the maze,” Nielsen explains.
Affordable, High-Volume Production
Although Orbital Composites can print high-tech products like satellite parts or antennas, one of its first customers is Lore, which offers custom-printed carbon shoes for bicyclists. Lore asserts that its shoes, which cost $1,900 a pair, will optimize a rider’s watt output and pedaling efficiency.
Using an iPhone app, customers scan their feet and send the measurements to the company, which uses Orbital Composites’ technology to print the shoes. Lore emphasizes the sustainability of its product. Their shoes are made from recyclable thermoplastics, and the printing process virtually eliminates waste.
“The shoes have demonstrated that Orbital Composites can cost-effectively manufacture a product using 12-axis, advanced thermoplastic, continuous fiber printing,” Nielsen says. Taking advantage of this technology, the company plans to manufacture and sell its own line of drones soon.
In addition, Orbital Composites is currently working on a project with the Department of Energy, ORNL and the University of Maine to 3D-print a wind turbine blade with continuous fiber. The goal is to demonstrate the feasibility of printing wind blades on site.
Another project with ORNL involves the development of additive manufacturing compression molding (AMCM).
“This particular machine is designed to produce thermoplastic continuous fiber and components with aerospace quality at the automotive price and production rate, with automotive production reliability and a Class A surface finish directly out of the machine,” says Amolak Badesha, Orbital Composites’ CEO. “There’s no material waste because the AMCM system prints the net weight and net shape of the part.” Badesha says that injection molding results in 10% waste, compression molding in 20% waste and sheet metal stamping in 55% waste.
“It’s really applicable to both car and airplane parts, and makes continuous fiber parts cheaper than sheet metal,” he adds. “That’s a first.”
Advances in Thermoset AM
From its founding in 2013, Massivit 3D has been focused on faster and more cost-effective manufacturing with large-scale 3D printing. Its printing system is unique; it uses a printing gel based on thermoset photopolymer resins.
“Most AM tooling systems for composite materials rely on a layering mechanism that utilizes thermoplastic materials,” says Ido Eylon, vice president of global sales and marketing. “This layering process causes uneven molecular bonds to form during the build process.”
The Massivit 10000, which will be commercially available in May, introduces the first isotropic 3D-printed mold to the composite manufacturing arena, according to the company. Leveraging a proprietary thermoset casting material, the technology allows for consistent and low thermal expansion at elevated temperatures, as well as high thermal stability up to 155 C.
The Massivit 10000, which won an Award for Composite Excellence at CAMX 2021, features a cast-in-motion technology. The printing head uses a rapid, UV-curing polymer to form two outer sacrificial walls, each about 3.6 millimeters thick. The casting head follows depositing an epoxy-based resin composite between those two walls, forming an isotropic core.
The printer builds up the mold layer-by-layer until the desired size and shape are achieved. (The maximum size is 56 inches wide, 59 inches high and 44 inches deep.) The hardened printed part, with the sacrificial walls still attached, can be post cured for a few hours to improve the mechanical properties at elevated temperatures, then immersed in water, where the outer walls flake off. (The flakes can be removed from the water, and the water reused.) The mold that comes out of the water is near net shape. The mold surface may require post finishing.
The process reduces the 19 steps typically required for mold production to just four, so companies using Massivit’s 3D printers will have their molds in less than a week rather than several weeks.
Some projects have been printed on the Massivit 10000, including a complex mold for a racing car seat. But there are many other potential applications. “All manner of tools and mandrels can be digitally produced for the transportation, home refurbishment, sports and aerospace industries,” says Eylon.
As additive manufacturing technology matures, companies will continue to find new and better ways to employ 3D printing on its own or in conjunction with other composite manufacturing techniques. The result should be faster, better and more cost-effective production methods that will provide the composites industry a competitive advantage in many markets.
Mary Lou Jay is a freelance writer based in Timonium, Md. Email comments to mljay@comcast.net.
########
An Additive Manufacturing Variation
Arris Composites’ Additive Molding™ technology isn’t 3D printing in the classic sense. “But the process is additive, because we are bringing subcomponents of different materials together to create a product,” says Riley Reese, the company’s CTO and cofounder.
Additive Molding is an automated process that begins with a continuous dry fiber impregnated with thermoplastic resin to create a filamentous material. This material is then shaped, using Arris’ proprietary process, into a 3D form that will be a component of the final part. The continuous fibers, which can be carbon, glass or aramid, are aligned along principal stress vectors.
The system then assembles the preformed shapes into a near net form that goes into the mold. Under the heat and pressure of the mold, the near net shape re-forms to the final part design. “We get a part that consistently meets expectations, and we also remove voids and porosity that would have existed without that molding step,” says Reese. “You also get incredible cosmetic surface finishes.”
Additive Molding also enables parts consolidation, resulting in single, multi-functional structures. For the Skydio X2 drone airframe, for example, Arris combined what had been 17 parts into one lighter-weight component with improved performance.
Skydio’s drone bracket made by Arris includes high-strength, stiff carbon fiber for the arms that hold and protect the optical equipment. The reinforcing carbon fibers at the ends of those arms are aligned to provide impact resistance. Glass fibers in the area around the drones’ GPS electronics create RF-transparent windows for unobstructed communication. The molding process enables Arris to provide both matte and glossy Class A surface finishes without the need for post-processing, Reese says.
Skydio had originally used 3D-printed titanium for its airframe. Replacing it with a consolidated composite frame provided the same stiffness and strength with an 80% reduction in weight.
With Additive Molding, Arris can reduce wall thicknesses in a composite part down to .25 millimeters, which opens up market opportunities in consumer electronics and consumer goods.
“Anything you want to carry around – your phone, a watch, sunglasses – all of these products can benefit from thinner and thinner walls that are structural,” Reese says. The process also enables the embedding of other functional components, such as a wireless charging coil, into structures like a cell phone case without added thickness.
The process of Additive Molding enables a production scale that 3D printing can’t match. “With a mold and a tool, we can get incredibly high volumes as well as a high level of repeatability and consistency,” says Reese. “Both of those things are challenges in the 3D printing world.”
SUBSCRIBE TO CM MAGAZINE
Composites Manufacturing Magazine is the official publication of the American Composites Manufacturers Association. Subscribe to get a free annual subscription to Composites Manufacturing Magazine and receive composites industry insights you can’t get anywhere else.